In an article on carrier phase based ranging, we saw how phase observations were employed to find the range between two wireless devices. Today we explore how phase can also be used for the purpose of location estimation. Background To determine the position of a wireless device, its range needs to be computed from a set of anchor nodes. When these anchors and the device itself are synchronized with each other, the signal propagation time of an electromagnetic wave arriving at these anchors after its emission from a Tx can be employed to calculate the corresponding distances. This is the
Continue reading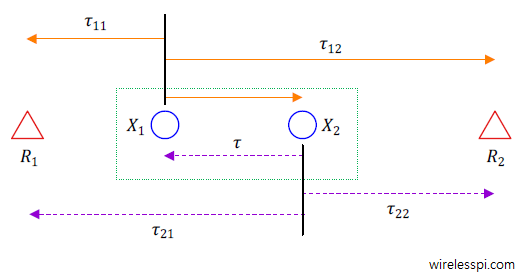